It starts with a breath—
the kind you take before a long walk,
or when you’re standing on the edge of something too big to name.
We are born into stories,
told how the world spins on its own,
how the sky will always be blue,
and the ground will always hold us steady.
But…
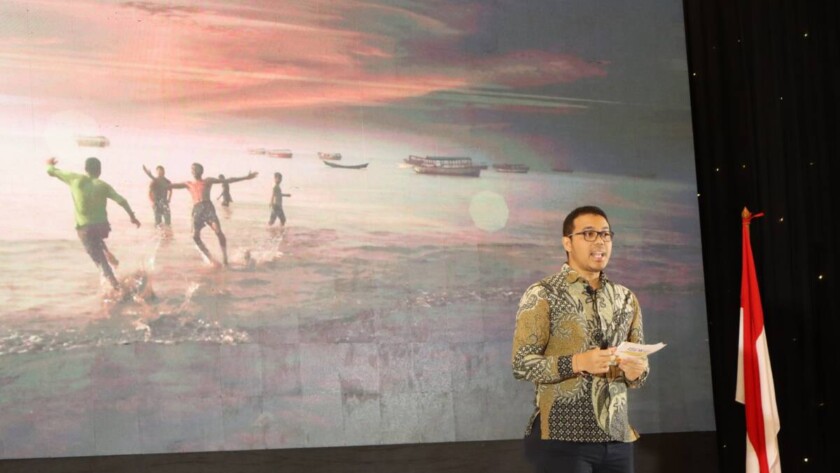